Using Matter Waves, Scientists Unveil Novel Collective Behaviors in Quantum Optics
Stony Brook University, November 25, 2024 -- A research team led by Dominik Schneble, PhD, Professor in the Department of Physics and Astronomy, has uncovered a novel regime, or set of conditions within a system, for cooperative radiative phenomena, casting new light on a 70-year-old problem in quantum optics.
Their findings on previously unseen collective spontaneous emission effects, in an array of synthetic (artificial) atoms, are published in Nature Physics ("Super- and subradiant dynamics of quantum emitters mediated by atomic matter waves"), accompanied by a theoretical paper in Physical Review Research ("Exact solution for the collective non-Markovian decay of two fully excited quantum emitters").
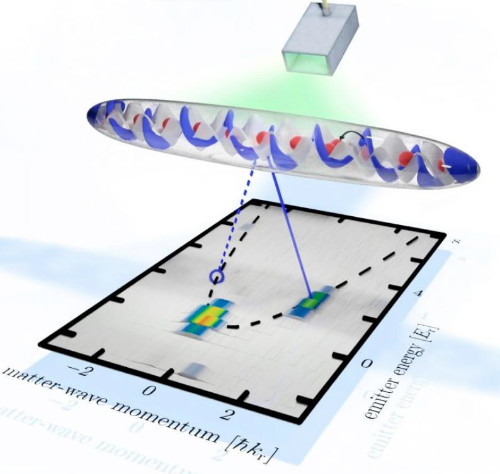
Spontaneous emission is a phenomenon in which an excited atom falls to a lower-energy state and spontaneously emits a quantum of electromagnetic radiation in the form of a single photon. When a single excited atom decays and emits a photon, the probability of finding the atom in its excited state falls exponentially to zero as time progresses.
In 1954 the Princeton physicist R. H. Dicke considered what happens when a second, unexcited, atom is put in its immediate vicinity. He argued that the probability of finding an excited atom would, surprisingly, fall to only one-half. The excited system consists of two simultaneous scenarios, one in which the atoms are in phase, leading to stronger emission (called superradiance), and one in which they are opposite in phase, when no emission occurs (subradiance). When both atoms are initially excited, the decay always turns superradiant.
Schneble and colleagues used a platform of ultracold atoms in a one-dimensional optical lattice geometry to implement arrays of synthetic quantum emitters that decay by emitting slow atomic matter waves. In contrast, conventional processes emit photons traveling at the speed of light. This difference enabled them to access collective radiative phenomena in novel regimes.
By preparing and manipulating arrays of emitters hosting weakly and strongly interacting many-body phases of excitations, the researchers demonstrated directional collective emission and studied the interplay between retardation and super- and subradiant dynamics.
“Dicke’s ideas are of great significance in quantum information science and technology (QIST). For example, there are intense efforts to harness super- and subradiance in arrays of quantum emitters coupled to one-dimensional waveguides,” says Schneble, also a member of Stony Brook’s Center for Distributed Quantum Processing (CDQP).
“In our work, we are able to prepare and manipulate subradiant states with unprecedented control. We can shut off spontaneous emission and observe where the radiation hides in the array. To our knowledge, this is a first such demonstration,” says Schneble.
The work by the Stony Brook team, which included two former PhD students Youngshin Kim and Alfonso Lanuza, provides new insights into some fundamental concepts of quantum optics.
Schneble explains that in Dicke’s theory, the photons do not play an active role since they move quickly between nearby emitters on the time scale of the decay. However, there are situations that can break this assumption, such as in a channel of a long-distance quantum network, where a guided photon escaping from a decaying emitter might need a long time to reach the neighboring one. This unexplored regime is exactly what the researchers were able to access because the emitted matter waves in their system are billions of times slower than photons.
“We see how collective decay from a superradiant state containing a single excitation takes time to form,” says co-author Kim. “It only happens once neighboring emitters have been able to communicate.”
The team points out that keeping track of slow radiation in a system of emitters is a daunting theoretical challenge.
Co-author Lanuza likens this challenge to a complicated game of catch and release: “A photon emitted by an atom can be caught back a few times before escaping, or even be bound to the atom. The rules of the game become complicated when multiple atoms and photons participate – atoms exchanging photons, photons bouncing off excited atoms, and photons getting trapped between atoms are just a few of the processes involved.”
Despite this complicated photon and atom interplay, he was able to find mathematical solutions for the case of two emitters with up to two excitations and arbitrary vacuum coupling. This aspect of the work may lead to uncovering other complicated or unexpected collective atomic decay behaviors in future experiments.
“Overall, our results on collective radiative dynamics establish ultracold matter waves as a versatile tool for studying many-body quantum optics in spatially extended and ordered systems,” concludes Schneble.