With Some Bumps, NIST Scientists Devise a Novel Way to Extend the Wavelength Range of Microcombs
Microcombs – chip-scale devices that generate and measure frequencies of light with exquisite accuracy – have transformed timekeeping and boosted optical communications. However, their operation is typically limited to a narrow range of near-infrared (NIR) wavelengths centered around 1,550 nanometers (nm), a wavelength often used for telecommunications.
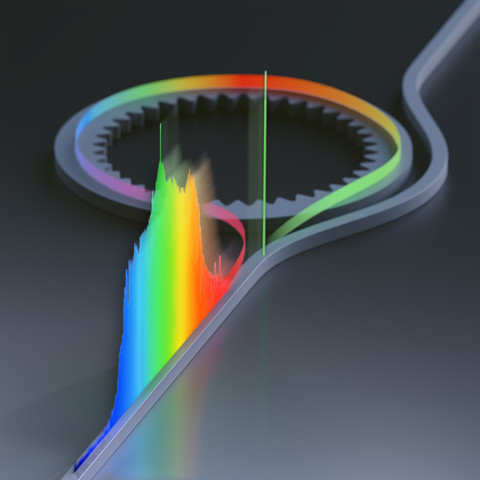
Now, researchers at the National Institute of Standards and Technology (NIST) and their colleagues have devised a novel way to extend the operating range of microcombs to shorter near-infrared wavelengths. The team’s method allows scientists to independently tailor the microcomb’s central wavelength as well as the intensity and separation of the frequencies it generates.
The shorter wavelengths of NIR light generated by the new frequency comb, centered around 1,064 nm, should prove a boon for probing biological samples. That’s because shorter wavelengths penetrate more deeply into biological tissues. (By comparison, visible light ranges in wavelength from 400 to 700 nm.)
Microcombs are usually powered by laser light injected into a microresonator – a ring-shaped racetrack that has a diameter smaller than that of a human hair. By carefully choosing the ring’s dimensions, laser light entering the ring can become a soliton – a solitary wave pulse that preserves its shape as it moves.
Each time the soliton completes one lap around the ring, a portion is siphoned off into a narrow channel called a waveguide. Ultimately, an entire train of the narrow pulses, fills the waveguide, with each pulse separated in time by the same interval – the time it took for the soliton to complete one lap. The sequence of pulses forms a set of evenly spaced, known frequencies that make up the tick marks, or “teeth,” of the comb, enabling the device to measure light waves of unknown frequency.
The exact color, range and number of spikes in the comb are determined by the optical properties and geometry of the microresonator, including the width and circumference of its ring. Researchers must choose a special combination of these properties to design a microcomb centered at the desired wavelength. This is a more challenging process for shorter near-infrared wavelengths, especially if researchers need to tailor the spectrum to meet the needs of particular experiments.
To create an NIR comb centered on 1064 nm and control the span of its teeth, researchers at NIST and the University of Colorado, Boulder, and their colleagues fabricated a microresonator so that the ring, which is ordinarily smooth, had tiny, periodic bumps or corrugations along its circumference. The corrugations transformed the resonator into a photonic crystal, a structure that guides and controls light at some frequencies and forbids light propagation at others, giving scientists added control over the frequency range of the microcomb.
By fine-tuning the width and spacing of the photonic crystal’s corrugations along with the dimensions of the ring, the team created a microcomb centered on the NIR wavelength of 1,064 nm. This is a significant step because it enables microcombs to be used in tandem with one of the most common and inexpensive lasers – those that emit light at 1,064 nm.
“Our work extends the capabilities of microcombs beyond the conventional telecommunication band, opening up new applications such as enhancing the interface between atoms and photonic chips and improving bioimaging and spectroscopy techniques,” said Grisha Spektor, who collaborated on the study while at NIST and the University of Colorado, Boulder. The new capability could enable scientists to take clearer images of biological and biomedical samples, and to study oxygen levels and metabolic rates in skin and muscle during exercise, added study collaborator Scott Papp of NIST.