Layered Hybrid Superlattices: A New Frontier in Designing Quantum Materials by Marrying Layered Crystals With Versatile Chemical Molecules
UCLA, November 21, 2024 -- A new paper titled “Layered hybrid superlattices as designable quantum solids” by Professor Xiangfeng Duan, postdoctoral researcher Dr. Zhong Wan and co-authors, recently published in Nature, introduces an exciting advancement in the development of customizable materials for quantum technologies.
First author Wan just recently received the prestigious UCLA 2024 Chancellor’s Award for Postdoctoral Research. He has been a postdoctoral research scholar at UCLA in the Department of Chemistry and Biochemistry, working in the Duan group since 2018.
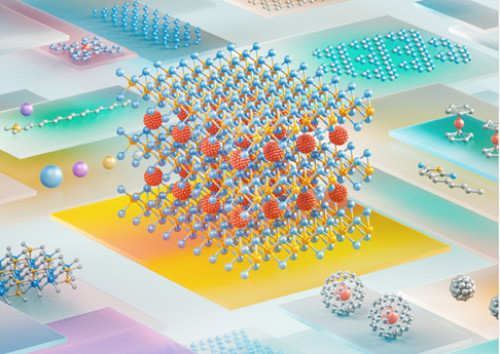
This perspective paper defined a new class of artificial solids called Layered Hybrid Superlattices (LHSLs). These materials bridge the gap between crystalline atomic solids and synthetic molecular systems, combining their strengths—long-range structural order and extensive tunability—while minimizing their individual limitations. LHSLs thus offer a modular and flexible framework for engineering new quantum properties, from superconductivity to chiral-induced spin selectivity, creating a promising platform for the next generation of quantum devices.
In traditional materials, designing quantum properties is limited by the strict chemical and structural compatibility needed to form heterostructures. However, LHSLs bypass these constraints by incorporating two-dimensional atomic crystals (2DACs), separated by customizable molecular interlayers. These interlayers create non-covalent interactions that allow a variety of atomic, molecular, and nanocluster species to be intercalated. This technique results in high-order superlattice structures with unprecedented flexibility in tailoring electronic, optical, and magnetic properties at the atomic level.
The authors summarizes different method of modular assembly in LHSLs, where the integration of distinct building blocks results in solids with versatile properties. This modular approach allows scientists to systematically design the electronic, optical, and magnetic landscape within these materials, forming structures with applications that range from room-temperature superconductors to quantum tunneling devices with tunable spin polarization. One highlighted application is in hybrid superconductors, where the addition of chiral molecular interlayers has been shown to produce unconventional superconductivity, which is a key area of interest for quantum computing.
The authors also emphasize the potential of LHSLs in creating a three-dimensional artificial potential landscape by combining 2DACs with molecular systems. This 3D periodic modulation opens the door to controlling low-energy excitations, offering new ways to study and exploit quantum behaviors like Bose-Einstein condensates and interlayer exciton superfluidity. By adjusting parameters such as interlayer spacing and chemical composition, LHSLs can create conditions for superfluid transition at much higher temperatures than traditional materials.
In summary, this paper lays the groundwork for a revolutionary approach to designing quantum materials, enabling a high degree of control over quantum properties and offering new functionalities beyond the current limits of material science. By achieving these advances, LHSLs have the potential to transform the field of quantum information and inspire a new class of devices and technologies. This publication represents a significant step forward in quantum material science, illustrating the synergy between chemistry, materials science, and quantum physics.
Corresponding author Professor Xiangfeng Duan joined the chemistry and biochemistry faculty in 2008. His group’s research interests include nanoscale materials, devices and their applications in future electronics, energy technologies and biomedical science.