Quantum Sensing Milestone Draws Closer to Exquisitely Accurate, GPS-Free Navigation
August 13, 2024 -- Now, scientists are attempting to make a motion sensor so precise it could minimize the nation's reliance on global positioning satellites. Until recently, such a sensor—a thousand times more sensitive than today's navigation-grade devices—would have filled a moving truck. But advancements are dramatically shrinking the size and cost of this technology.
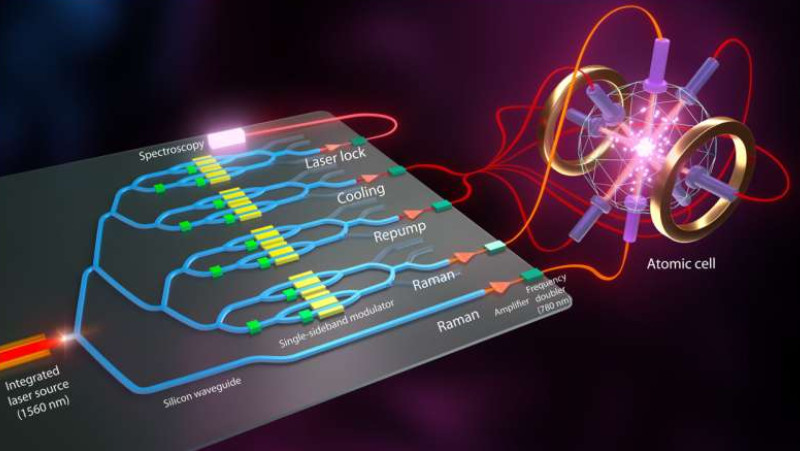
For the first time, researchers from Sandia National Laboratories have used silicon photonic microchip components to perform a quantum sensing technique called atom interferometry, an ultra-precise way of measuring acceleration. It is the latest milestone toward developing a kind of quantum compass for navigation when GPS signals are unavailable. The team published its findings and introduced a new high-performance silicon photonic modulator—a device that controls light on a microchip—as the cover story in the journal Science Advances.
"By harnessing the principles of quantum mechanics, these advanced sensors provide unparalleled accuracy in measuring acceleration and angular velocity, enabling precise navigation even in GPS-denied areas," said Sandia scientist Jongmin Lee.
Typically, an atom interferometer is a sensor system that fills a small room. A complete quantum compass—more precisely called a quantum inertial measurement unit—would require six atom interferometers.
But Lee and his team have been finding ways to reduce its size, weight and power needs. They have already replaced a large, power-hungry vacuum pump with an avocado-sized vacuum chamber and consolidated several components usually delicately arranged across an optical table into a single, rigid apparatus.
The new modulator is the centerpiece of a laser system on a microchip. Rugged enough to handle heavy vibrations, it would replace a conventional laser system typically the size of a refrigerator. Lasers perform several jobs in an atom interferometer, and the Sandia team uses four modulators to shift the frequency of a single laser to perform different functions. However, modulators often create unwanted echoes called sidebands that need to be mitigated.
Sandia's suppressed-carrier, single-sideband modulator reduces these sidebands by an unprecedented 47.8 decibels—a measure often used to describe sound intensity but also applicable to light intensity—resulting in a nearly 100,000-fold drop.
Besides size, cost has been a major obstacle to deploying quantum navigation devices. Every atom interferometer needs a laser system, and laser systems need modulators. Miniaturizing bulky, expensive components into silicon photonic chips helps drive down these costs.
And since they can be manufactured using the same process as virtually all computer chips, "This sophisticated four-channel component, including additional custom features, can be mass-produced at a much lower cost compared to today's commercial alternatives, enabling the production of quantum inertial measurement units at a reduced cost," Lee said.
As the technology gets closer to field deployment, the team is exploring other uses beyond navigation. Researchers are investigating whether it could help locate underground cavities and resources by detecting the tiny changes these make to Earth's gravitational force. They also see potential for the optical components they invented, including the modulator, in LIDAR, quantum computing and optical communications.
The team's grand plan—to turn atom interferometers into a compact quantum compass—bridges the gap between basic research at academic institutions and commercial development at tech companies. An atom interferometer is a proven technology that could be an excellent tool for GPS-denied navigation. Sandia's ongoing efforts aim to make it more stable, fieldable and commercially viable.