New Photonic Chip Spawns Nested Topological Frequency Comb
Scientists on the hunt for compact and robust sources of multicolored laser light have generated the first topological frequency comb. Their result, which relies on a small silicon nitride chip patterned with hundreds of microscopic rings, will appear in the June 21, 2024 issue of the journal Science.
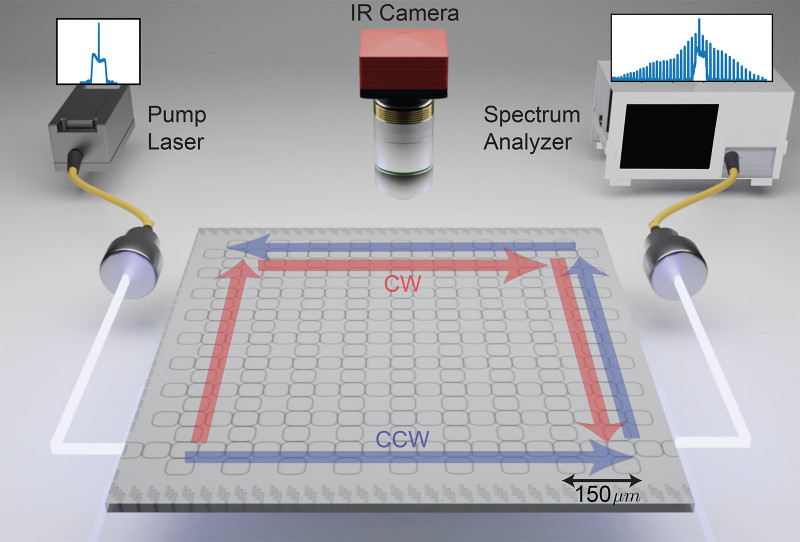
The earliest frequency combs required bulky equipment to create. More recently researchers have been focused on miniaturizing them into integrated, chip-based platforms. Despite big improvements in shrinking the equipment needed to generate frequency combs, the fundamental ideas haven’t changed. Creating a useful frequency comb requires a stable source of light and a way to disperse that light into the teeth of the comb by taking advantage of optical gain, loss and other effects that emerge when the source of light gets more intense.
In the new work, JQI Fellow Mohammad Hafezi, who is also a Minta Martin professor of electrical and computer engineering and physics at the University of Maryland (UMD), JQI Fellow Kartik Srinivasan, who is also a Fellow of the National Institute of Standards and Technology, and several colleagues have combined two lines of research into a new method for generating frequency combs. One line is attempting to miniaturize the creation of frequency combs using microscopic resonator rings fabricated out of semiconductors. The second involves topological photonics, which uses patterns of repeating structures to create pathways for light that are immune to small imperfections in fabrication.
By designing a chip with hundreds of resonator rings arranged in a two-dimensional grid, the research team engineered a complex pattern of interference that takes input laser light and circulates it around the edge of the chip while the material of the chip itself splits it up into many frequencies. In the experiment, the researchers took snapshots of the light from above the chip and showed that it was, in fact, circulating around the edge. They also siphoned out some of the light to perform a high-resolution analysis of its frequencies, demonstrating that the circulating light had the structure of a frequency comb twice over. They found one comb with relatively broad teeth and, nestled within each tooth, they found a smaller comb hiding.
Although this nested comb is only a proof of concept at the moment—its teeth aren’t quite evenly spaced and they are a bit too noisy to be called pristine—the new device could ultimately lead to smaller and more efficient frequency comb equipment that can be used in atomic clocks, rangefinding detectors, quantum sensors and many other tasks that call for accurate measurements of light. The well-defined spacing between spikes in an ideal frequency comb makes them excellent tools for these measurements. Just as the evenly spaced lines on a ruler provide a way to measure distance, the evenly spaced spikes of a frequency comb allow the measurement of unknown frequencies of light. Mixing a frequency comb with another light source produces a new signal that can reveal the frequencies present in the second source.
At least qualitatively, the repeating pattern of microscopic ring resonators on the new chip begets the pattern of frequency spikes that circulate around its edge. Individually, the microrings form tiny little cells that allow photons—the quantum particles of light—to hop from ring to ring. The shape and size of the microrings were carefully chosen to create just the right kind of interference between different hopping paths, and, taken together, the individual rings form a super-ring. Collectively all the rings disperse the input light into the many teeth of the comb and guide them along the edge of the grid.
The microrings and the larger super-ring provide the system with two different time and length scales, since it takes light longer to travel around the larger super-ring than any of the smaller microrings. This ultimately leads to the generation of the two nested frequency combs: One is a coarse comb produced by the smaller microrings, with frequency spikes spaced widely apart. Within each of those coarsely spaced spikes lives a finer comb, produced by the super-ring. The authors say that this nested comb-within-a-comb structure, reminiscent of Russian nesting dolls, could be useful in applications that require precise measurements of two different frequencies that happen to be separated by a wide gap.
While iterating on the chip design, Flower and his colleagues also discovered that it would be difficult to deliver enough laser power into the chip. In order for their chip to work, the intensity of the input light needed to exceed a threshold—otherwise no frequency comb would form. Normally they would have reached for a commercial CW laser, which delivers a continuous beam of light. But those lasers delivered too much heat to the chip, causing them to burn out or swell and become misaligned with the light source. They needed to concentrate the energy in bursts to deal with these thermal issues, so they pivoted to a pulsed laser that delivers its energy in a fraction of a second.
The team says that while their experiment is specific to a chip made from silicon nitride, the design could easily be translated to other photonic materials that could create combs in different frequency bands. They also consider their chip the introduction of a new platform for studying topological photonics, especially in applications where a threshold exists between relatively predictable behavior and more complex effects—like the generation of a frequency comb.