Untangling the Entangled: Quantum Study Shines Fresh Light on How Neutrinos Fuel Supernovae
June 21, 2024 -- Researchers used quantum simulations to obtain new insights into the nature of neutrinos — the mysterious subatomic particles that abound throughout the universe — and their role in the deaths of massive stars. The study relied on support from the Quantum Computing User Program, or QCUP, and the Quantum Science Center, a national Quantum Information Science Research Center, at the Department of Energy’s Oak Ridge National Laboratory.
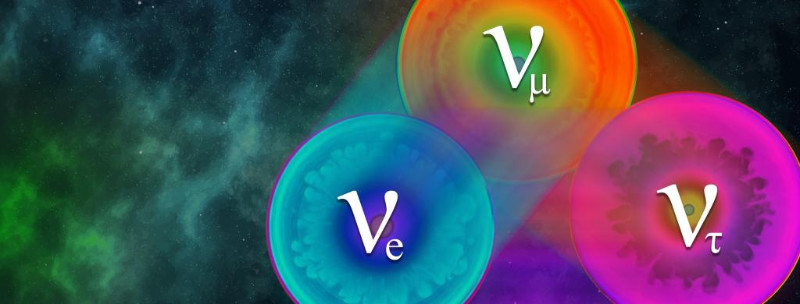
“This understanding was something new that hasn’t come out of classical computing systems,” said Martin Savage, the study’s senior author and a professor of physics at the University of Washington. “We recognized for the first time we could study how entanglement between multiple neutrinos is induced over time, and these results are within the error bars of what we’d expect from a classical computer. It’s a step in the direction of better, more accurate and more scalable quantum simulations.”
Neutrinos result from nuclear reactions — from the huge reactions that cause the sun to shine, to the tiny reactions that enable radioactive tracers for medical tests. These extremely light particles appear everywhere, carry no electric charge and seldom interact with other matter. But during the collapse and explosion of a star — a process better known as a supernova — neutrinos exchange energy and momentum with not just each other but with everything around them.
“These neutrinos are entangled, which means they’re interacting not just with their surroundings and not just with other neutrinos but with themselves,” Savage said. “It’s extremely difficult to simulate this kind of system, because entanglement’s an intrinsically quantum-mechanical property beyond what we can capture and approximate in classical computing. That’s why we need a quantum computer that uses calculations based on quantum physics to model what’s happening.”
Savage and his co-author Marc Illa of the University of Washington’s InQubator for Quantum Simulation obtained an allocation of time on Quantinuum’s H1-1 quantum computer via QCUP, part of the Oak Ridge Leadership Computing Facility, which awards time on privately owned quantum processors around the country to support research projects. The Quantinuum computer uses trapped ions as qubits, one of several quantum computing approaches.
Quantum computers store information in qubits, the quantum equivalent of bits. Qubits, unlike classical bits, can exist in more than one state simultaneously via quantum superposition – more like a dial with a wider range of more detailed settings than an on/off switch. That difference enables qubits to carry more information than classical bits. Scientists hope to use this increased capacity to fuel a quantum computing revolution built on a new generation of devices.
That capacity allowed Savage and the research team to simulate an approximation of the quantum-mechanical interactions between a supernova’s neutrinos. An actual supernova would involve a minimum of a septendecillion, or 1054, neutrinos. Savage and Illa began their simulation using a simpler model with a system of 12 neutrinos.
Quantum circuits – the quantum equivalent of traditional digital circuits – allowed the team to model the complicated connections and interactions between the particles so that each neutrino could interact with each of the others, not just its nearest neighbors.
The results offered a realistic approximation of how neutrinos become entangled at the quantum level, so that changing the properties of one also changes the properties of another. During a supernova, neutrinos can change flavor from an electron flavor to a muon flavor or to a tau flavor as the neutrinos begin to interact with each other and their surroundings. The detail provided by the simulations enabled the team to measure the evolution from one flavor to another over time of various entangled neutrinos.
Why track the flavor conversion? Because the mu and tau flavors of neutrinos interact differently with matter than their electron-flavored brethren. These interactions can impact the amounts and types of heavier elements produced in the supernova explosion.
“These circuits turned out to approximate the neutrinos’ behavior very well,” Savage said. “We discovered we could use these simulations to measure neutrino entanglement in a statistically significant way and that we could identify a significant scaling in size as the number of neutrinos increased. This was the first time this kind of study had been done.”
The primary hurdle for useful quantum simulations has been the relatively high error rate caused by noise that degrades qubit quality. The problem’s so common the current generation of quantum computers has become known as noisy intermediate-scale quantum, or NISQ. Various programming methods can help reduce these errors, but Savage and Illa didn’t need those methods to conduct their study thanks to the high quality of the Quantinuum computer’s qubits and gates. The computer’s 12-qubit circuits proved to be sufficient for almost 200 of the 2-qubit gates.
“We found the systematic errors on the quantum hardware were less than the statistical errors,” Savage said. “We still have a long way to go to predict the behavior of large neutrino systems with precision, and we don’t know whether the current generation of NISQ devices can take us there. But this technique should be portable to other types of quantum computers, and the results help us set protocols that can be used to simulate larger systems of neutrinos.”